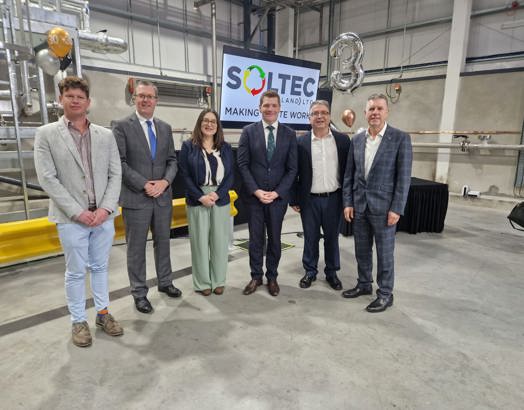
01 Oct 2024
10 minutes to read
Mission-critical infrastructure is the lifeblood of our interconnected world. These complex assets, which include data centres (DCs), hospitals, airports, etc. are indispensable in maintaining the smooth functioning of all aspects of our daily lives.
DCs for instance power our digital communications and store vast amounts of data, ensure the operational efficiency of healthcare systems and facilitate global travel, and ultimately uphold the machinery of 21st century democratic governance.
However, the operation of mission-critical infrastructure leads to significant energy consumption. Wherever a continuous and reliable power supply is required to function effectively, often the price is remarkably high energy demand and resultant Carbon emissions. The growing Carbon footprint of mission-critical facilities, particularly those associated with DCs is a major concern and requires significant efforts to achieve decarbonisation.
These pressing issues have necessitated the exploration of more sustainable solutions to enhance the operational efficiency of these infrastructures, while significantly reducing their environmental impact. Among the various solutions being explored - and one that holds considerable promise - is the use of microgrids.
Microgrids for Mission-Critical Infrastructures
Microgrids are small-scale power grids that can operate and be controlled independently or in conjunction with the main electrical grid. They are gaining prominence due to their versatility, ability to adopt to location-specific renewable resources, use of local e- and bio-fuel supplies and ultimately addressing the citizen and industrial needs at much lower overall Carbon emissions.
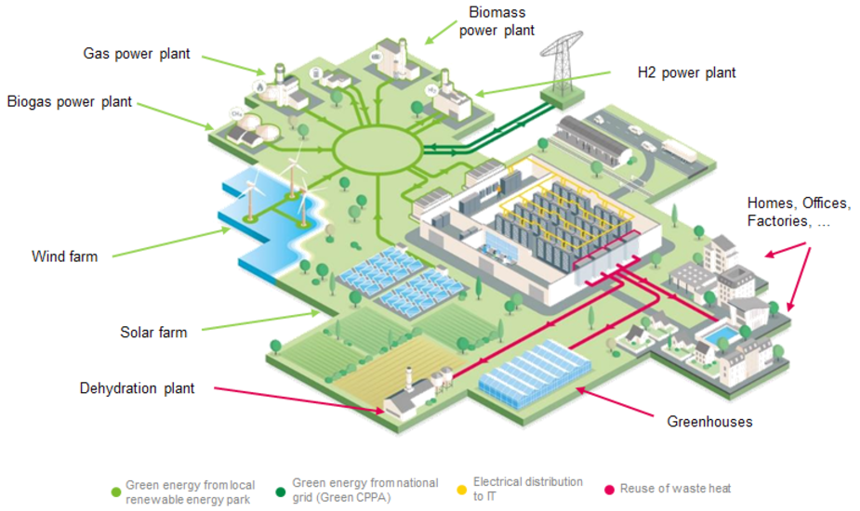
Figure 1. Schematic of a notional microgrid based on renewable energy sources connected to a data centre [1]
They also provide resilience by operating independently of the main grid, which is crucial for infrastructures where downtime can be costly. Furthermore, they can provide grid support services, resulting in a closer relationship between the infrastructure, the hosting community and local utility providers.
While the initial CapEx of a microgrid may be significant, the long-term cost savings, resilience, local employment benefits, and environmental impact make them a worthwhile investment.
Organic Rankine Cycle (ORC) in Microgrids
The Organic Rankine Cycle (ORC) is a thermodynamic cycle that converts heat into mechanical energy, which can then be converted into electricity. This process is particularly effective for low-grade heat sources, such as waste heat from an industrial process or building systems [2]. ORCs use organic fluids with low boiling points and typically requires input temperatures between 150°C and 350°C [3], and in instances as low as 100°C [4]. Heat is the system input and electrical power is output, with the operating process divided into four phases [5]:
- Pressurising the working fluid and pumping it into an evaporator.
- Boiling the working fluid into a vapour using an external heat source (still within the evaporator).
- Expanding the vapour in an expander attached to a generator to produce electricity.
- Condensing the vapour back into a fluid to repeat the cycle.
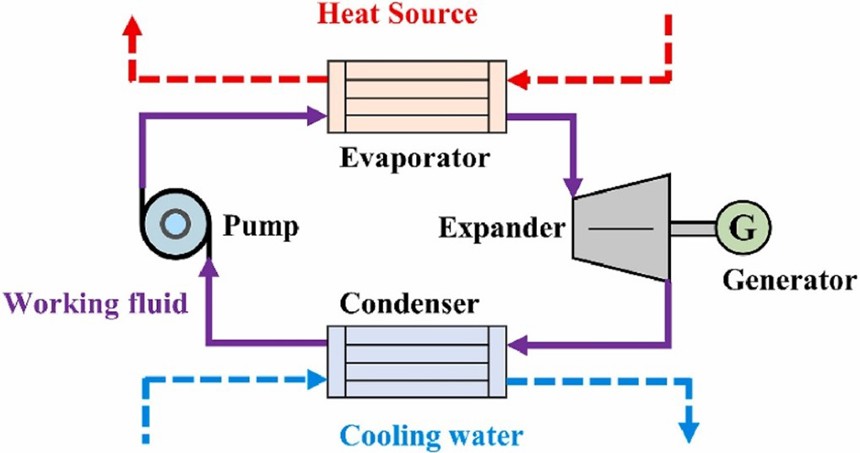
Figure 2. Schematic of Organic Rankine Cycle (reproduced from [3])
ORC systems have been successfully implemented in a wide range of applications. Early advances focused on waste heat recovery (WHR) in industrial applications, internal combustion engines, and gas turbine exhausts. More recent research has explored the use of ORC systems with renewable energy sources such as biomass, concentrated solar systems, and geothermal energy [6].
Despite the promising potential of ORC systems, their overall efficiencies remain quite modest, and their cost can be prohibitive. However, essentially their input is waste heat that would otherwise be discarded and the need for heat reuse in modern microgrids can increase their implementation [6].
At larger scales associated with utility level outputs (i.e. multi-MW), ORCs are well-established power generation assets [7]. However their adoption in the 1–100 kW range is limited to an estimated total installed capacity of approximately 4.95 MW worldwide [8].
Integrating ORC with Microgrids
Mission-critical infrastructures, such as DCs, airports, and hospitals, can greatly benefit from ORC integration into their microgrids. The benefits are enhanced energy efficiency, improved reliability, and environmental credentials. The quantities of waste heat in DCs (with continuous generation) are growing to be particularly alarming and harnessing this heat for power generation could present significant opportunities.
It is possible to quantify the benefit of ORCs with a careful and case-by-case balance of techno-economic considerations, evaluation of ORC technology type, working fluid characteristics, grade, quantities of waste heat, system resilience, and Total Cost of Ownership. RED Engineering Design is working on a concept design in which ORCs will be integrated into a scalable, geographically agnostic microgrid for mission-critical applications. The next image illustrates an overview of how we envisage the ORC as a part of our future microgrid templates.
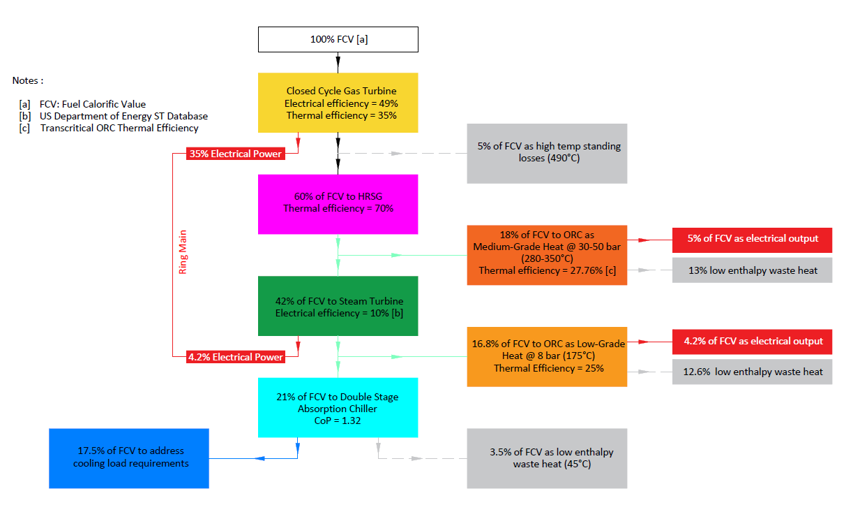
Figure 3. RED’s geographically agnostic microgrid template with the integration of ORC system
Here, and at a steady state mode in which gas turbines (in the first of the two combined cycles) are at the most optimum operating point, 35% of the total fuel calorific value (CV) will be converted to electrical power, while 5% will be the standing loss through the turbine body and its exhaust. The remaining 60% of the fuel CV will be directed to a Heat Recovery Steam Generator (HRSG). The HRSG will recover 42% of the remaining fuel CV in the form of steam that is fed to a steam turbine to generate additional electricity (realising another 4.2% of original fuel CV as power). 18% of fuel CV (now in the form of medium-grade heat at 280°C -350°C) will be in excess of steam turbine intake and would otherwise be released as waste heat. It is at this stage that transcritical ORCs come in to enhance overall system efficiency. We will be able to utilise 18% of fuel CV and realise another 5% of it as valuable electricity.
Furthermore, 16.8% of the fuel CV will come out of steam turbine at too low an enthalpy to be useful and would need to be discarded. Once again, a second, low-temperature ORC system can come in at this stage and convert an additional 4.2% of the Fuel CV to electricity. It must be noted that in thermodynamics, the calorific value of fuel, and the ability of electrical power to do useful work (compared to low- and medium-grade heat) does not make a direct comparison between these energy vectors easy. It is therefore for illustrative purpose that we treat fuel and its thermo-electrical derivatives homogeneously in Fig 3.
Another interesting consideration is that most multi-utility microgrids need to be optimised for power or cooling output depending on the utility demand of the client and time of year. For instance in the template summarised in Fig 3, when additional cooling is required, double-stage absorption chillers are prioritised with ORC processing any additional turbine thermal output. At time of low cooling demand, the microgrid is optimised for power production with ORC processing maximum amount of thermal energy available. The steady state process in Fig. 3 is a single point of performance in which double-stage absorption chillers will use 21% of total fuel CV to address cooling load requirements, and release 3.5% of fuel CV as waste heat at a low temperature of 45°C. This clearly illustrates the value of ORCs in increasing generational flexibility of microgrids and fully valorising fuel CV.
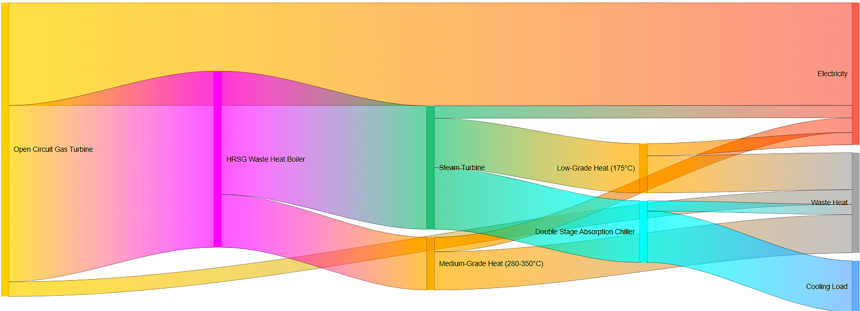
Figure 4. Sankey diagram of a CCGT-based microgrid driving absorption chillers and a cascade of ORC systems
Fig 4 summarises how the integration of advanced ORCs can boost the overall electrical efficiency of the microgrid from 49% to 58.4%. Low- and medium-grade heat do carry substantial amounts of enthalpy which at multi-MW scales, and when the asset-based is designed for continuous production across its lifetime, should be fully exploited.
Conclusion
Integrating microgrids with Organic Rankine Cycle (ORC) technology offers a promising path toward sustainable and efficient energy use in hyperscale mission-critical infrastructures. Microgrids that are designed to support industrial clusters involving DCs are likely to have magnitudes of scale where ORC become techno-economically feasible. In addition to enhanced overall fuel utilisation by valorising waste heat, ORCs add flexibility to a multi-utility microgrid where the system output can be optimised for power or cooling. While these benefits are substantial, a case-specific evaluation remains crucial. RED continues to assess ORC’s roles in some of its large-scale template designs to unlock new opportunities for waste heat recovery and overall system efficiencies.
Our initial findings show that utilising ORC in a microgrid can significantly improve energy efficiency and operational flexibility. At steady state condition and at times of low cooling demand, 18% of the total fuel CV is recovered at medium-grade heat (i.e. 30-50 bar, 280-350°C) and 16.8% of total fuel CV at heat at low-grade (i.e. 8 bar, 175°C). These thermal resources, when combined with ORCs with a maximum reported conversion efficiency of 27.76% [9], will increase the power output of our microgrids by 9.2%, utilising surplus heat that otherwise would have been rejected to the environment.
Sources:
- ENGIE. (2022, July 26). Retrieved from https://www.engie.com/en/campaign/green-data-centers
- Miftah Hijriawan, H. A. (2022, January). Organic Rankine Cycle (ORC) system in renewable and sustainable energy development: A review of the utilization and current conditions for small-scale application. Journal of Applied Engineering Science. doi:10.5937/jaes0-36319
- Xiaolei Damon Yuan, Y. L. (2023). Waste heat recoveries in data centers: A review. Renewable and Sustainable Energy Reviews. doi:10.1016/j.rser.2023.113777
- Saleh, Bahaa, et al. "Working fluids for low-temperature organic Rankine cycles." Energy 7 (2007): 1210-1221.
- Obi, J. B. (2015, Decmber). State of Art on ORC Applications for Waste Heat Recovery and Micro-cogeneration for Installations up to 100kWe. Energy Procedia, 82, 994-1001. doi:10.1016/j.egypro.2015.11.857
- Radulovic, J. (2023). Organic Rankine Cycle: Effective Applications and Technological Advances. Energies, 16(5), 2329. doi:10.3390/en16052329
- Sylvain Quoilin, V. L. (2009). Technological and economical survey of organic Rankine cycle systems. 5th European Conference Economics and Management of Energy in Industry.
- ORC World Map. (n.d.). Retrieved from - https://orc-world-map.org/
- Guo C, Du X, Yang L, Yang Y. Performance analysis of organic Rankine cycle based on location of heat transfer pinch point in evaporator. Applied Thermal Engineering. 2014;62(1):176-186
Latest Insights
View all insights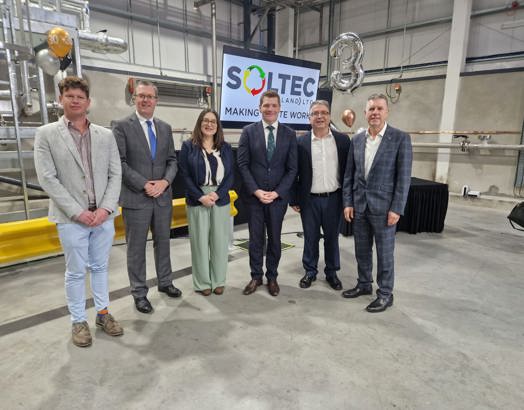
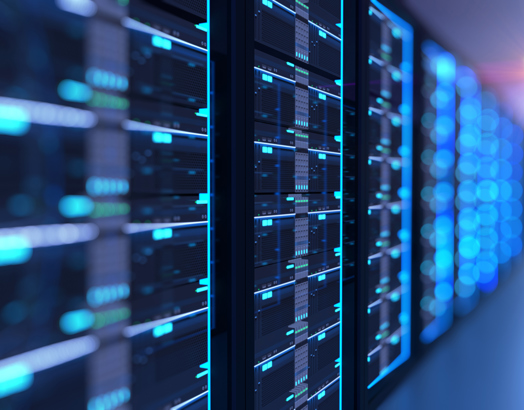
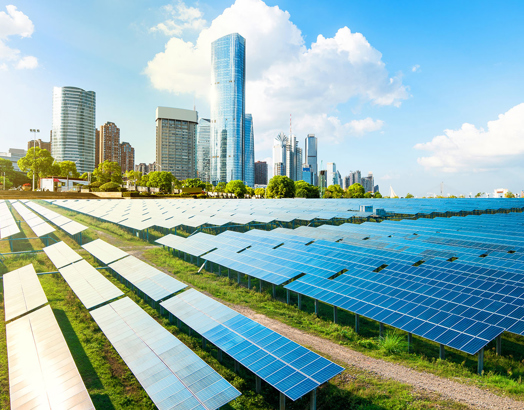
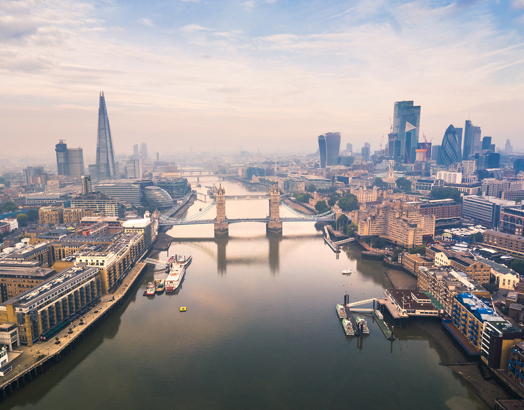
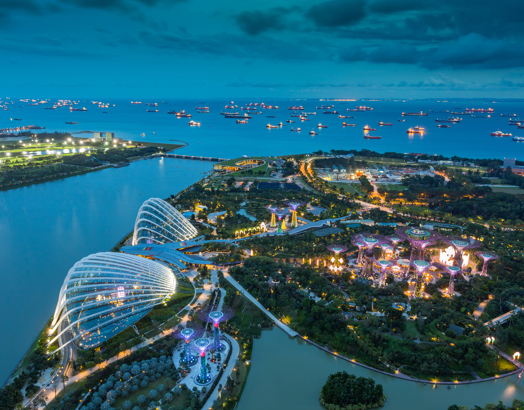