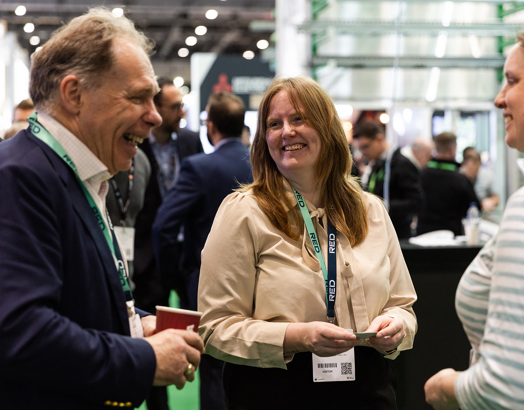
13 Dec 2024
10 minutes to read
As climate change intensifies, engineers are increasingly driven to innovate new methods for energy extraction, distribution and utilisation.
The energy sector is pivotal to achieving global targets for Carbon emission reduction, making the evolution of heating and cooling systems a critical area of focus. This evolution has led to a greater centrality of district energy networks which can act as the integration platform for smart local energy systems and have gained increasing prominence over the past few decades as a solution for heating and cooling of residential and commercial consumers.
Over the years, the design of District Heating and Cooling Networks (DHCNs) has evolved from the 1st to the 5th generation, with 3rd and 4th generation networks being the most commonly utilised today. 3rd generation DHCNs distribute heat from decentralised plants to end-users through a buried water network that extends to the boundary of the consumer’s building, typically at supply temperatures ranging from 60°C to 90°C. In contrast, 4th generation networks improve energy efficiency by operating at lower supply temperatures of 45°C to 60°C and use higher levels of insulation [1]. Within the consumer buildings, the internal network delivers heat to meet the requirements for space heating and domestic hot water.
DHCNs provide a more cost-effective and environmentally friendly alternative to traditional centralised heating and cooling systems [2]. Consequently, government initiatives in the UK and EU have introduced new guidelines, energy strategies, and funding incentives to support their wider implementation [3,4,5].
DHCNs also offer the advantage of utilising a diverse range of previously overlooked heat sources, such as geothermal, waste heat from data centres (DCs) and other municipal and industrial activities. Recent technological advancements have made it possible to harness these resources effectively, paving the way for more sustainable energy solutions.
District cooling networks are less established in the market apart from parts of the Middle East, and have until recently been primarily developed for commercial consumers [6]. As demand for cooling escalates due to the unprecedented growth of DCs [7] and with the electricity grid transitions decarbonisation, 5th generation energy networks emerge as an increasingly attractive solution for achieving a net-zero Carbon approach to heating and cooling systems in energy-dense areas. However, the commercial viability of these networks requires a case-by-case evaluation.
5th generation heating & cooling networks (5G DHC)
The topology of a 5G DHC network can vary depending on the integrated technologies and operational philosophy. Nonetheless, all methodologies are based on the fundamental principle of circulating water at low temperatures throughout a flow and return header running around the site, serving as a source or sink for heat. Individual heat pumps at the building or end-user level then generate the necessary temperatures for space heating and cooling , as well as domestic hot water. Heat is extracted from the network to meet heating demands or rejected back into the network during cooling periods [8]. When demand is unbalanced, decentralised plants that integrate renewable heat sources and utilise the ground for storage can effectively address deficiency or excess of thermal energy within the network [9].
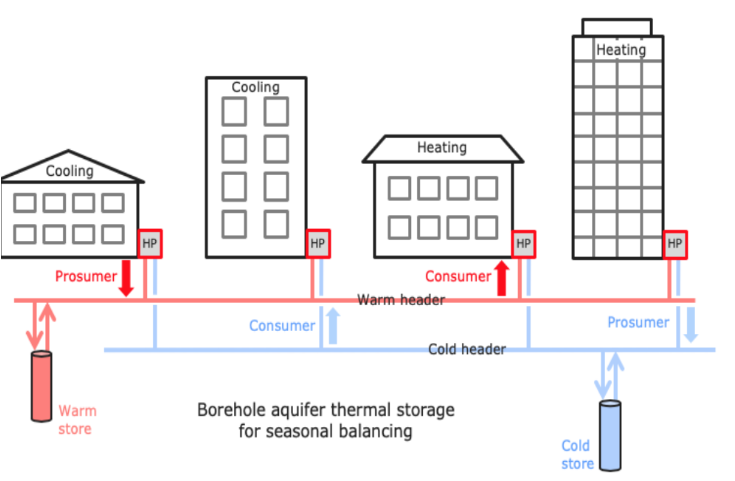
Figure 1. 5th generation heat network with flow and return headers [8].
The network architecture of 5G DHC system depends on the variables associated with its components and design parameters. The two primary topologies are ambient loop networks and ultra-low temperature networks.
The ambient loop topology operates usually at a temperature range of 10˚C to 30 ˚C (e.g. 25 ˚C flow / 15 ˚C return), whilst the ultra-low temperature is configured at temperatures of <45˚C on the flow header and a return header at a range of 15-25˚C [8].
The cooling process heat rejection serves as an input to meet heating demand. This means balancing the interconnected loads are essential. Utilising ground aquifers can address this challenge, particularly when heat sources are available but do not align with the demand operating profile. Separate Aquifers (warm / cool) can provide seasonal below-ground thermal storage for both heating and cooling, making it possible to use the stored energy in these aquifers as and when needed [9].
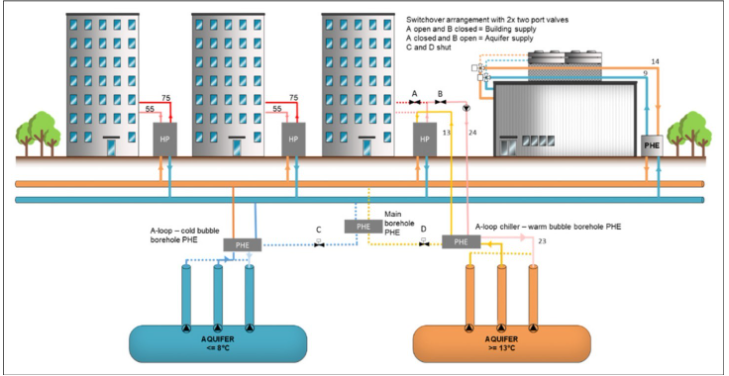
Figure 2. Concept of 5GDHC network with use of aquifers [9].
Benefits & challenges of 5G DHC
The design of 5G DHC networks is still subject to ongoing research and development, with various concepts implemented in pilot projects within the market with a greater development seen in Switzerland and Germany [2]. Several factors influence the architecture of a 5G DHC system, including the operating temperatures of cooling systems, the availability of nearby cooling processes, end-user technologies, the feasibility of utilising the ground for storage, reliability requirements, and the scale of connected consumers. Examples of these 5G DHC topologies are illustrated in Figure 3 below.
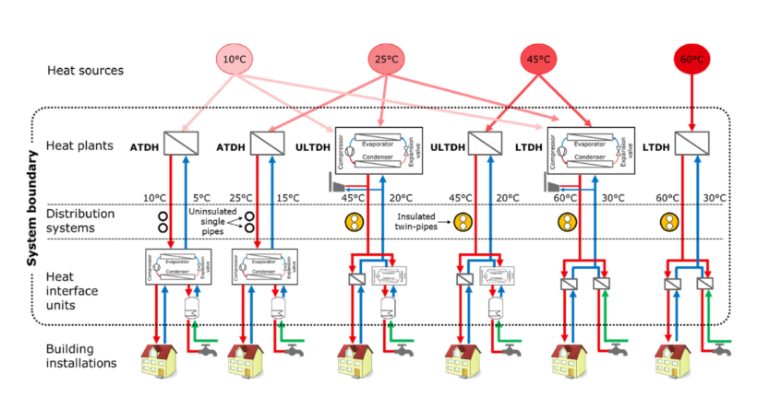
Figure 3. Concept layout of a 5GDHC networks operating at ambient, ultra-low and low temperatures [6].
The networks shown in figure 3 are categorised as follows:
- ATDH: for Ambient Temperature District Heat;
- ULTDH: Ultra Low Temperature District Heat, and
- LTDH: Low temperature District Heat.
Studies have shown that different 5G DHC concepts can present varying economic profiles. Integrating aquifers or below-ground formations as energy storage can significantly enhance the economics of 5G DHC networks. However, the associated drawback is the upfront CapEx incurred for geothermal surveys, excavation and infrastructure integration. A desktop study can provide some initial feasibility insights [9].
In the case of ambient loops, the very low operating temperatures lead to drastically minimised heat losses, thereby reducing the need for insulation. Reduced insulation results in lower CapEx (studies have shown that CapEx can be reduced by up to 40% compared to insulated pipes of the same dimensions [6]). However, with lower network supply temperatures, the heating mode heat pump efficiency in the connected buildings will also go down.
Other challenges include the spatial requirements for integrating heat pumps and local thermal storage at the building and end-user level within existing developments. Enhancements in energy centre designs and the use of phase change materials in thermal storage can help address issues related to spatial constraints [9].
The major advantage of 5G DHC networks lies in their ability to utilise heat from integrated cooling processes, which improves the efficiency of heat pumps. Without this integration (e.g. 4th Generation concept), heat pumps would need to extract heat from the environment, where temperatures are typically low and fluctuate broadly. 5G DHC provides the heat pumps with a much tighter and more favourable performance envelope which improves their operating efficiencies, with lower operational costs and substantial Carbon emissions reductions.
From a purely economic perspective, relying on electrically powered heat pumps as the primary technology in the system can impact the project's economics, making a 5G DHC solution more challenging compared to a 3rd and 4th Generation network, particularly in countries where electricity prices are significantly higher than those of gas [9].
In addition, 5G DHC infrastructure costs remain high compared to 4th Generation networks that make it challenging to arrive at a compelling techno-economic case without detailed analysis. One example is the larger pipework required in 5G DHCN as a result of the smaller difference in flow and return temperatures when compared to the 4G version. To address this, innovative business models, an integrated and well executed energy masterplan and long-term commitments are essential [9]. These models could incorporate smart grid principles that couple power generation with thermal supply to a community, incorporating a diverse set of technologies such as photovoltaics (PVs), wind farms, Hydrogen plants, solar thermal systems, and most importantly waste heat recovery from DCs.
A family of tools developed by ENGIE such as BE Circle and NEMO can evaluate the viability of heat networks focussing on key factors such as Carbon footprint, cost-effectiveness, and sustainability.
Waste heat from DCs
The efficiency of heat pumps is a critical factor in calculating a project's economics. Thus, the supply temperature to end-users and the available temperatures from cooling prosumers are essential components of this equation. DCs utilise cooling processes that, depending on the system type, can provide waste heat at various temperatures. In typical air-cooled systems, waste heat can be available at up to 25-30°C, while liquid cooling systems can achieve even higher temperatures (around 45°C or possibly higher [10]). Integrating this waste heat into a 5G DHC system can minimise the energy contribution required from heat pumps to meet energy demand of end-users. However, implementing 5G DHC in existing buildings with high energy demand in the absence of fabric energy efficiency is challenging. Otherwise heat pumps capable of high temperature outputs would be needed [6].
The waste heat generated by DCs is consistently available throughout the year. In contrast, the demand for heating and hot water follows a variable seasonal and diurnal profile, with peaks occurring during the day. This means the thermal energy available from a DC can only be utilised when needed. Hence thermal storage including those in aquifers, below ground formations and surface vessels become an indispensable consideration to address the challenge of unbalanced loads within the network [9].
Conclusion
5G DHC systems present a promising solution for a sustainable future in the energy sector. Careful consideration is needed in initial design phases to identify appropriate heat sources, such as DCs, in order to enhance system efficiency and commercial viability. Developing a system that embraces a holistic approach to power generation and heat supply within a community is a major master-planning challenge. However by effectively integrating DC waste heat into a 5G DHC network, considerable Carbon emissions both for the DC and the wider energy system is possible. With careful masterplanning, involvement of all appropriate stakeholders, and long term and mutually beneficial contracts, 5G DHC can be framed into a competitive business model.
References
[1] Phil Jones, Paul Woods, Martin Crane, Chartered Institution of Building Services Engineers (CIBSE) and Association for Decentralised Energy (ADE), 2020, “Heat networks: Code of Practice for the UK, CP1 Second Edition”.
[2] Simone Buffaa, Marco Cozzinia, Matteo D’Antonia, Marco Baratierib, Roberto Fedrizzia, 2019, “5th generation district heating and cooling systems: A review of existing cases in Europe”, 08 February, journal homepage: www.elsevier.com/locate/rser, https://doi.org/10.1016/j.rser.2018.12.059
[3] Department for Energy Security and Net Zero website, 2024, “Heat Networks Delivery Unit: Round 14”, Retrieved from https://www.gov.uk/guidance/heat-networks-delivery-unit (online)
[4] European Commission Directive 2012/27/EU (Energy Efficiency Directive), 2021, “Article 14: Potential for efficiency in heating and cooling”, Retrieved from https://energy.ec.europa.eu/document/download/7f7b7b1a-d7c1-447f-a18b-cd1fefa8af50_en?filename=BE-WAL%20CA%202020%20en.pdf (online)
[5] Lord Callanan, 2023, “Thousands of homes to be kept warm by waste heat from computer data centres in UK first”, Retrieved from https://www.gov.uk/government/news/thousands-of-homes-to-be-kept-warm-by-waste-heat-from-computer-data-centres-in-uk-first (online)
[6] Oddgeir Gudmundsson, Ralf-Roman Schmidt, Anders Dyrelund, Jan Eric Thorsen, 2021, “Economic comparison of 4GDH and 5GDH systems - Using a case study”
[7] Dame Dawn Childs (DCD website), 2024, “The wicked problem delivering data center capacity in an uncertain world”, https://www.datacenterdynamics.com/en/opinions/the-wicked-problem-delivering-data-center-capacity-in-an-uncertain-world/ (Online)
Latest Insights
View all insights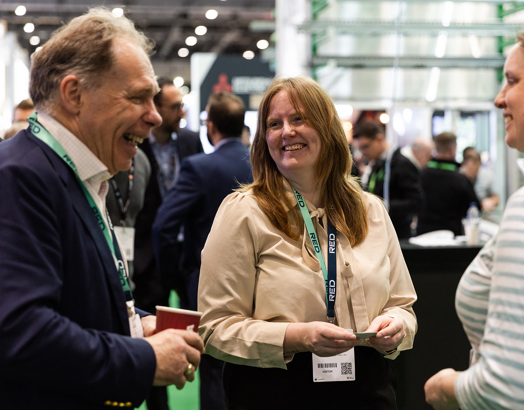
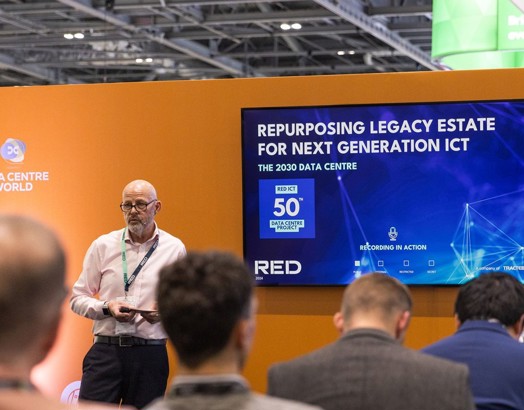
Safeguarding Tech Investments in the Age of AI: Insights from RED ICT
11 Feb 2025
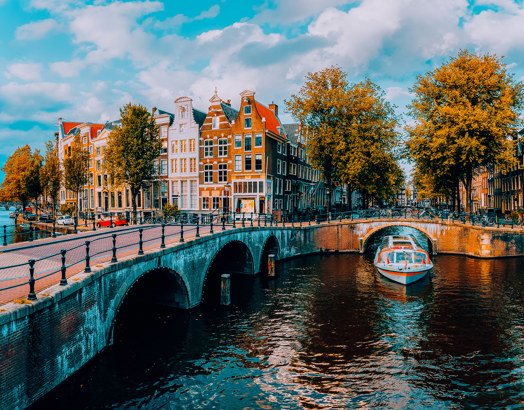
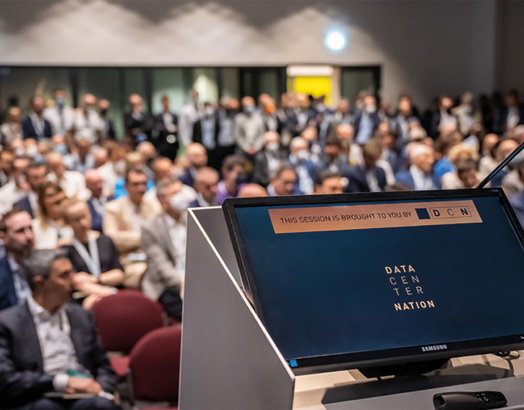
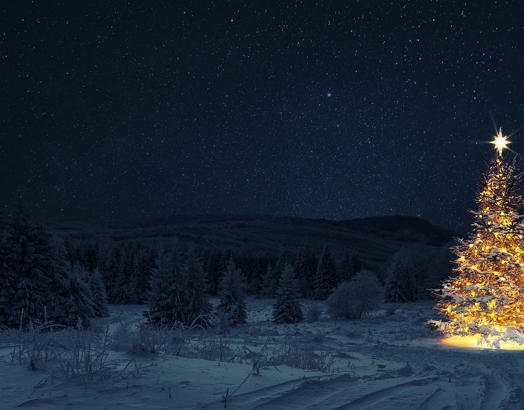
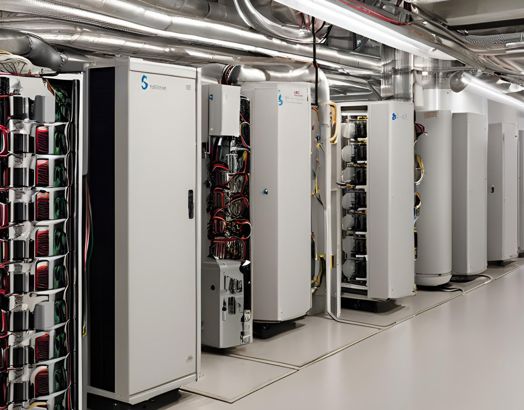
5th Generation Heat Networks: Enhancing System Level Efficiencies By Integrating Data Centre Waste Heat
13 Dec 2024